What Is Kelvin In Lighting? Colour Temperature (K) Explained
When looking at different light options it is highly likely that you’ve come across lights with different Kelvin values. Some standard Kelvin values for most commonly used lighting are 2700K, 3000K and 4000K.
In this article, we will explain what these numbers mean, how it is measured and some of the science behind what makes this aspect of lighting important.
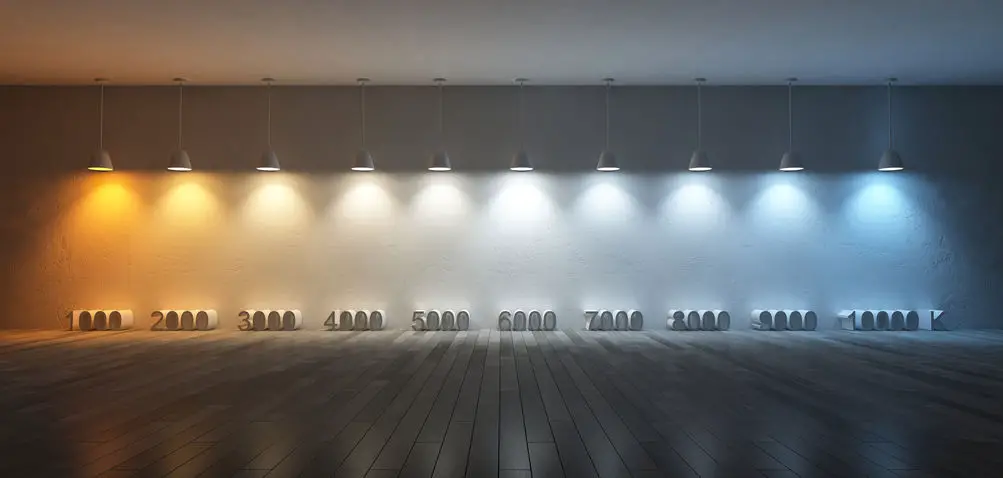
Contents
What is Kelvin In Lighting?
Kelvin (K) in lighting refers to the colour temperature of any specific light source. Colour temperature is defined as the visual temperature a blackbody radiator has when heated to specific Kelvin temperatures. Common blackbody radiators are incandescent light bulbs and the sun.
What this means in practicality is that lights with a low K value will appear warm in orange/yellow tones whereas lights with a high K value will appear white/blue. The logic and reasons for this are explained under the subheading Blackbody Radiators Explained.
How Kelvin Is Measured
As mentioned, Kelvin for lights is a scale that is used to describe lights via their colour temperature.
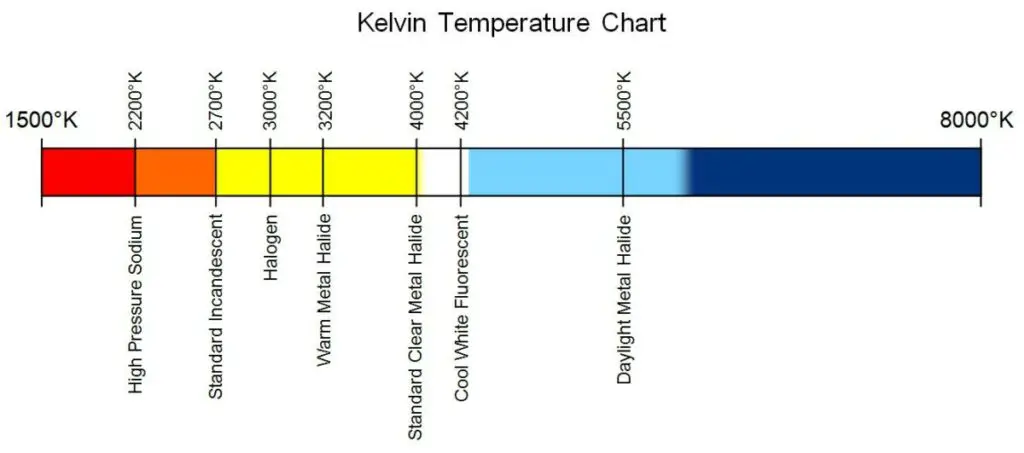
Blackbody Radiators Explained
To fully understand how Kelvin is measured we need to understand the basics of blackbody radiators and blackbody radiation.
The term “blackbody radiator” is defined in physics as a light source whose light spectral radiation is directly linked to its thermal temperature.
In a more practical way of identifying blackbody radiators, you can think of them as light sources that produce light as a result of heat generation or by burning natural fuels. A few examples of light sources that fit the blackbody radiator criteria are:
- Fire
- Candlelight
- Incandescent bulbs
- Halogen bulbs
- The sun
While all these light sources work slightly differently from each other they all function based on the same principles, which allows all of them to be classed as blackbody radiators.
Since these lights are all blackbody radiators it also means that all of their colours are directly impacted by the temperature of the light source.
To envision how this works we can look at candle fire. When lighting a candle it emits a very warm orange flame most of the time. This flame (depending on the candle) is normally around 1000 °C (1832 °F), which works out to be roughly 1300K in Kelvin.
The conversion formulas between the different temperature scales work out to this:
- Kelvin to Celsius: 0K − 273.15 = -273,1°C
- Celsius to Farenheit: (0°C × 9/5) + 32 = 32°F
- Farenheit to Kelvin: (32°F − 32) × 5/9 + 273.15 = 273,15K
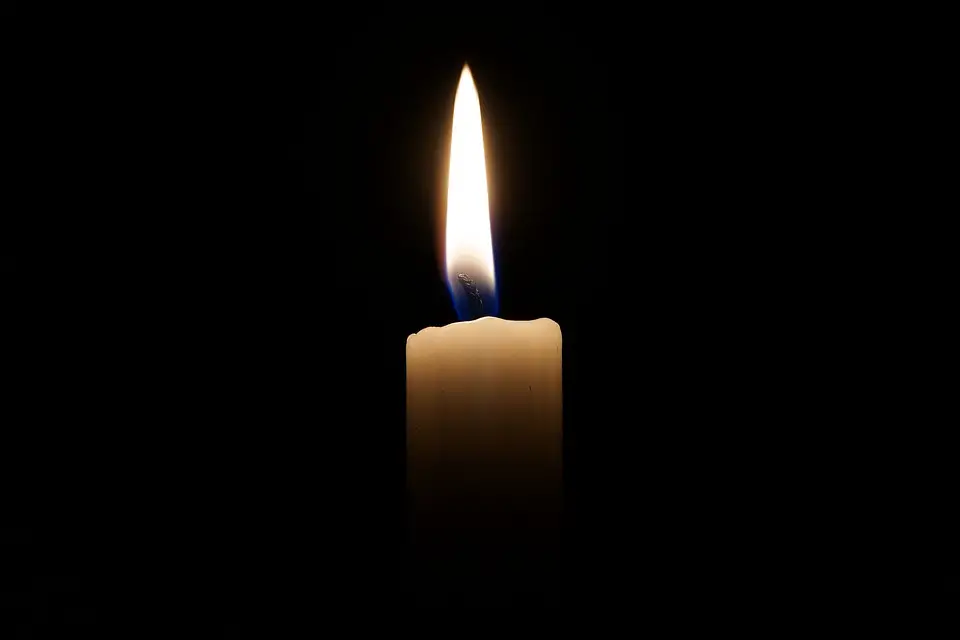
While the normal state of candlelight is around 1000 °C, it would begin to change colour if the flame was hotter. If we hypothetically were to take a candle and get the flame to reach a temperature of 6000 °C it would be the equivalent of 6200K, which means the flame would actually be rather blue due to the intense heat.
It is obviously unrealistic to get a candle to reach that sort of temperature, but theoretically, that is what would happen if it ever reached that temperature.
Another way to think about this is that at the very bottom of a candle flame it is a slight shade of blue, due to the fire closest to the burning wick being the hottest part of the flame.
This is also why you rarely see lights with a K value less than 1000K. It is simply because blackbody radiators require a higher temperature than that to produce any form of visible light to begin with.
Lights with a lower Kelvin than 1000K mostly produce light in the infrared spectrum, which is essentially just considered heat emission in this context.
Planck’s Law
To fully grasp how the Kelvin scale works we need to dive into the realm of physics a bit. For this, we need to discuss Planck’s law of radiation.
Planck’s law of radiation effectively describes the electromagnetic radiation a blackbody radiator gives off at any given temperature. This is essentially what we have been describing above when talking about blackbody radiation, but Planck’s law offers some expanded depth to the science by making it possible to plot it in a chromaticity diagram.
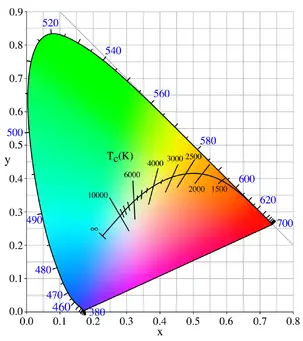
This right here is a chromaticity diagram. It is a commonly used diagram that plots every colour into a 2D space using the wavelengths of visible light.
Around the middle of it, there is a black line with a few numbers marked along it. This is the natural curve that blackbody radiators follow when reaching these Kelvin temperatures according to Planck’s law.
This is an extension of Planck’s law which is often referred to as “Planck’s locus”. Using this it is easy to see how the Kelvin temperature will affect the perceived colour of the light.
White light
Another important aspect of Planck’s law to consider is white light. White light is normally defined within physics as a light that contains all wavelengths of visible light at equal intensity.
This can be achieved artificially with LED technology by having 3 equally strong red, blue and green diodes emit light together. However, a section of blackbody radiation is also considered white.
The section of blackbody radiation that can be considered white light is found at Kelvin temperatures of 4000-6000K. At this point in Planck’s locus, the curve goes through a section of the chromaticity diagram where all the colours converge and create a pure white light. Therefore, this section appears white when viewed in real light sources.
Now we have discussed how light sources that follow blackbody radiation fit into the Kelvin scale, but how do light sources that don’t fit into this category get graded? For this, we have to turn to correlated colour temperature.
Correlated Colour Temperature (CCT) is the Colour Temperature (K) value given to light sources that don’t follow natural blackbody radiation. This value is determined by the Kelvin value of the reference point on Planck’s locus that closest resembles the light source being graded.
To explain this more thoroughly, we need to take another look at the chromaticity diagram. Along the main blackbody radiation curve, there are lines running perpendicular to it with their respective Kelvin values noted next to them. These are the lines that help us grade non-blackbody lights.
All light sources have a place in this diagram, regardless of their origin or scientific method of light creation. Even if they are placed a far bit away from Planck’s locus they will still receive a CCT (K) number thanks to these perpendicular reference lines.
The way these lines work is that they act as reference points throughout the diagram. In a practical sense, they are used to simply see which point in Planck’s locus the artificial light has the closest relation to.
CIE 1931 Colour Spaces
Everything discussed regarding CCT so far is based on “CIE 1931 Colour Spaces”. CIE is the International Commission on Illumination who in 1931 invented a system known as “CIE 1931”.
CIE 1931 is a system that manages to pinpoint any light source’s position in the chromaticity diagram. The math used to determine this is rather complex, but the results of it are CIEx and CIEy values.
These are the values you can apply to the chart, where CIEx gives you the X-coordinate for the light and CIEy gives you the Y-coordinate. In order to understand this better, I would recommend you check out Waveformlighting.com’s CCT calculation tool to get a visual representation of how this works.
While CCT is a good method of giving artificial lighting a Kelvin value it has a catch. It is good for artificial lighting that is relatively close to Planck’s locus, but not so great for lights further away.
The reason for this is that the further out from Planck’s locus you go the light colour rapidly becomes monochromatic.
So hypothetically, you could have a light that emits a monochromatic green light but still have it be rated as 5000K because the 5000K point in the diagram was the closest to the monochromatic green light. This can cause the scale to become inaccurate and unreliable.
However, there is a way to be weary of this caveat. A monochromatic green light would score extremely poorly from a CRI standpoint.
CRI is short for Colour Rendering Index, which is a grade given to all light sources. This grade is between 0-100CRI and is much like Kelvin based on blackbody radiation and the sun. Click here for a more elaborate explanation of the Colour Rendering Index.
To explain this point simply, a monochromatic green light would score a CRI of around 5-10, which means the light quality of that light source is extremely poor. So, by taking both Kelvin and CRI into consideration the CCT system works because lights below CRI60 rarely hit the market anyway.
Properties Of Different Kelvin Values
After having discussed how different K values are achieved and how it is measured in the first place, is there actually any noticeable difference between them other than the fact that we see them as different colours?
Cool vs Warm Light
At this point, we need to make a distinction in the Kelvin scale and that distinction is where we draw the lines for warm and cool light. Generally speaking, it is broken down like this:
- Extra Warm: 2000-2700K
- Warm White: 2700-3500K
- Cool White: 3500-6000K
- Daylight: 6000K
- Cold white: 6000K+
With these distinctions in place, we can more easily talk about the different points of colour temperature and compare them.
Perceived Feeling
One of the main differences between warmer whites and cooler whites is the perceived feeling we get when subjected to them.
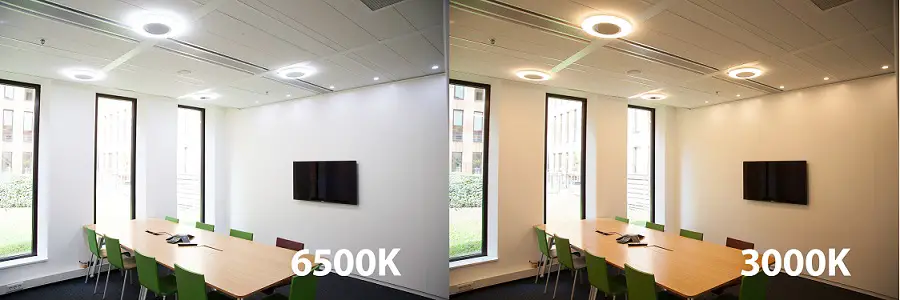
Cool whites make you feel awake. They will make you more focused and concentrated. They also give off a more “clean” and sterile feeling. This is why buildings such as hospitals make it a point to use cool white lighting because they need to be perceived as clean.
Warm whites make us feel relaxed. The warmth of warm white lights can be comparable to open fire, they make you feel warm and cosy. This is why warm white lights are often used in home environments, they simply make a home feel more like home.
Differentiating Between Different Colour Temperatures
While this point is not necessarily a property of colour temperature itself, it is relevant to how we perceive them.
This property has to do with how the human eye is mostly unable to identify a light’s colour temperature.
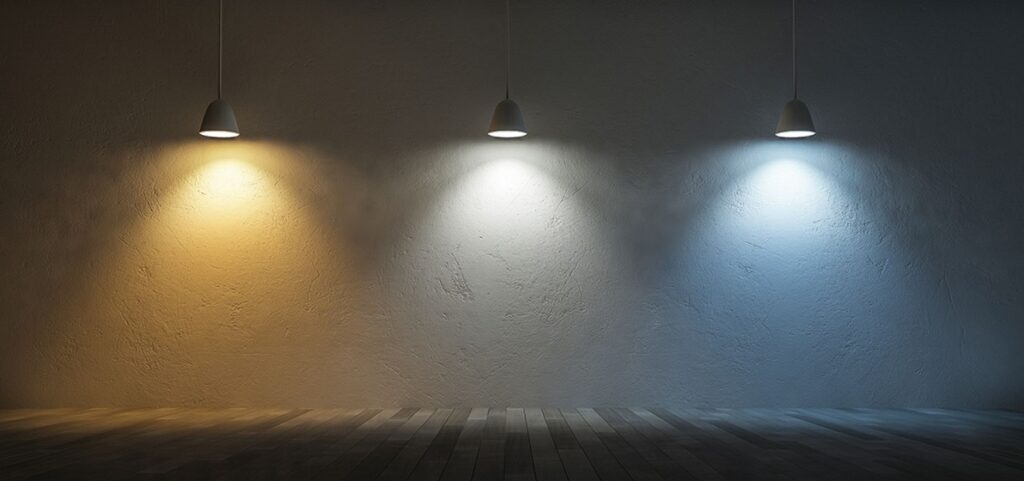
If we are shown a light at 3000K and are asked to identify what colour temperature it is, a lot of people would be unable to tell the colour temperature correctly.
This is because in order for us to assess a light’s colour temperature we need to have another reference point next to it.
A very well-trained eye can recognize colour temperature fairly accurately, but we are not biologically trained to spot this.
Physiological Effects
The reason why we feel certain things with different Kelvin values of light is that there is a direct link between the colour temperature in light and our biological programming.
The reason for this has to do with the sun. Over the course of a day, the sun changes its colour temperature. During dusks and dawns it will have a colour temperature of around 2000-2500K while it peaks at around 6000K at its highest point during the day.
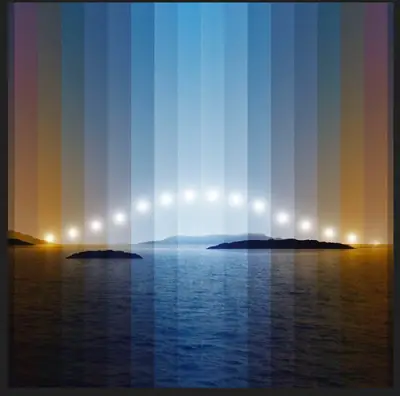
The reason this happens has to do with the way sunlight reflects on our atmosphere when it looks at earth from different angles.
At the sun’s highest point most of the visible light enters the atmosphere, which causes it to appear as a cooler white. However, when the sun is positioned towards our horizon it causes most of the blue wavelengths to “scatter” when it hits the atmosphere.
All of this ties into the Circadian rhythm, which essentially describes the “natural body time” for people. This is all linked together because the circadian rhythm is more or less based on the sun’s colour temperature due to the sun being the basis for all living life on earth.
Melatonin is our body’s sleep hormone. Melatonin growth is promoted in our body when exposed to warm light and is suppressed when subjected to cool light. This is the exact reason why cool lights are used in buildings such as schools and offices.
Kelvin Rating Among Common Bulbs
Now that we have discussed the properties of colour temperature we can take a look at the colour temperature of some common light bulb types.
Light Bulbs | Colour Temperature |
---|---|
Incandescent | 2700K |
Halogen | 3000-3400K |
CFL | 2700-4000K |
LED | 2200-20000K |
Low-Pressure Sodium | 2200K |
Mercury Vapour | 3600K |
Some of these bulbs have a fixed colour temperature. This simply has to do with the construction of the bulbs.
Incandescent bulbs operate at a temperature of around 2500 °C (4600 °F) and cannot go higher than that since the basic construction can’t handle that much heat. This is why halogen bulbs can reach a colour temperature of up to 3400K.
They use the exact same light generation principle as incandescent bulbs, but because they can run at a hotter temperature of around 2700 °C (4892 °F) they manage to emit a cooler light. This ties back together with the fact that both are blackbody radiators. Click here to read more about the heat generation of different lights.
Some of these bulbs also have ranges, mainly fluorescent-based lighting and LED lighting. This is simply because the way they are constructed allows there to be a large variety in their artificial light qualities.
Summary
To summarize, here are the most important takeaways from this article:
- Colour Temperature is measured in Kelvin, which itself is a measurement based on the actual thermal temperature of blackbody radiators such as the sun, fires and incandescent bulbs.
- There are different portions of colour temperature that all bring different aesthetical and physiological effects when used.
- Correlated Colour Temperature (CCT) is used for lights that don’t follow blackbody radiation, such as LEDs and Fluorescent lighting.